Solutions for carbon-intensive industries
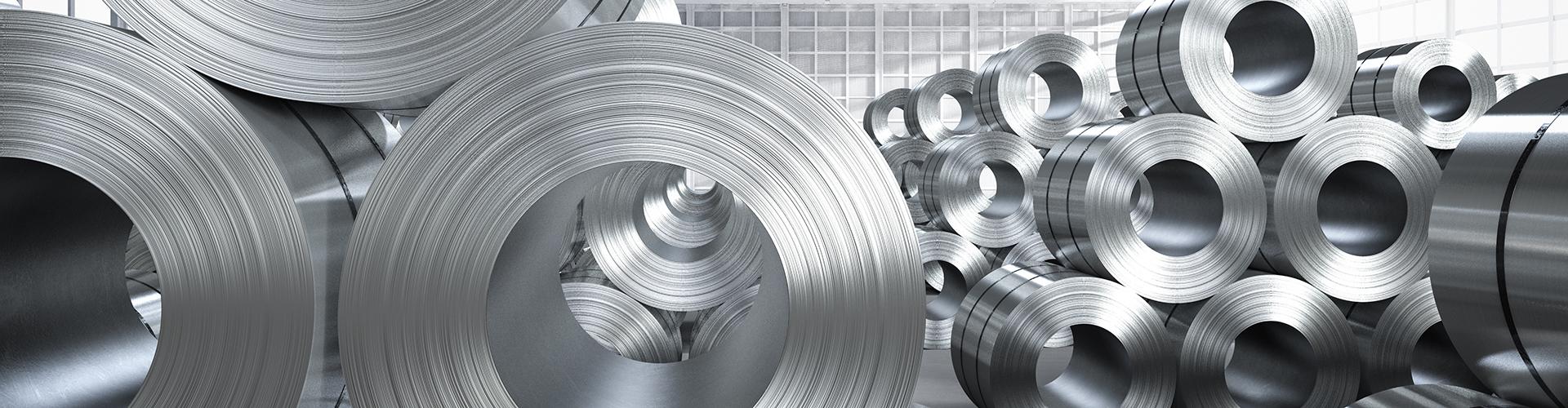
High carbon-intensity industries
Future clean energy systems will need to supply low-carbon energy and enable greater energy efficiency in end-use applications across the board, whether in electricity and heat production for industrial and domestic use, in agriculture, forestry and other forms of land use or in transportation.
Among the main sectors, heavy industry is particularly carbon intensive and its emissions are particularly hard to abate. According to the International Energy Agency, heavy industry's direct CO2 emissions in the G7 countries alone amount to around 6 billion tonnes per year, more than one-sixth of total CO2 emissions from the global energy system. Given that the global steel and cement industries are each responsible for at least 8% of global equivalent carbon emissions, we explore the innovations taking place to make these two key industries cleaner and more sustainable.
- Green steel
-
Iron is produced from iron-oxide mineral ore in blast furnaces, using red-hot coke and carbon-containing gases to reduce the ore to iron and iron carbide, releasing CO2. The raw iron is further refined to remove excess carbon and achieve the desired grade of steel. All this requires a huge amount of energy: overall, roughly two tonnes of CO2 are emitted for each tonne of iron produced.
According to IPCC studies, the steel industry is responsible for approximately 8% of global CO2 emissions. Research institutions and the steel industry itself are investing in measures to reduce emissions. One promising new steel production process involves the use of hydrogen as a reducing agent instead of carbon-containing reductants; ideally this should be green hydrogen using electrolysis from renewable energy.
Further possibilities lie in the use of renewable energy sources throughout the production process, more heat recycling, the re-use of scrap steel and the optimisation of existing processes.
Avoiding, capturing or reforming CO2
Avoiding CO2Avoiding or reducing CO2 emissions in the production process must remain the primary goal. Optimising the use of alternative reducing agents to replace the current CO2-generating coke is a priority, the primary candidate being hydrogen (see dedicated smart search below). However, other possible reducing agents are being explored, such as readily available methane.
Capturing or reforming CO2
Where it is not possible to avoid CO2 emissions, the capture and storage or reforming of CO2 offer an alternative. CO2 can also be converted into carbon monoxide for re-use in the steel furnace.
Hydrogen as reducing agent
Steel production traditionally uses coke as a carbon source to reduce the iron oxide ore, resulting in enormous CO2 emissions. If hydrogen is used as an alternative - preferably hydrogen produced via electrolysis powered by renewable wind or solar energy - the only by-product is steam.
Hydrogen as reducing agent - full result set
Process efficiency and heat recovery
There are various ways of improving the overall efficiency of current steel production processes. Optimisation of operating conditions and more energy-efficient furnaces can be complemented by enhanced heat recovery and re-use. The steam produced can serve to generate electricity by conventional means, and the top gases from the furnace can be used for heating other processes. To avoid having to reheat the newly produced steel, it can be immediately used in a subsequent process such as steel rolling, before it is allowed to cool down.
Co-generation with other technologies
Co-generation focuses on the re-use of CO2 in other technologies. This involves modifying or cleaning the emitted CO2, in dedicated scrubbers, for example, and its subsequent re-use in other chemical or biochemical processes (or a combination of both). Examples of such processes include using microbes to produce methane.
Co-generation with other technologies - full result set
Recycling scrap in electric furnaces
High-quality steel can be produced directly from scrap steel in an electric furnace, or scrap steel can be included as a raw material in conventional steel-making furnaces. Processes to facilitate these techniques are being continually refined and optimised.
- Green cement
-
Cement is a binder material that is combined with sand and gravel or other aggregates to produce concrete. Concrete itself is the most widely used material today, excluding water. Hydraulic cement, mostly Portland cement, sets and forms a hardened matrix through hydration to produce interlocked silicate hydrates, and is particularly resistant to weather-induced and chemical erosion.
Cement kilns are traditionally heated using coal or petroleum coke. The kilns have a very high energy demand as the raw materials, including limestone (calcium carbonate), have to be heated to around 1 450°C. Deacidification of the limestone also releases CO2. Global cement production currently stands at around four billion tonnes per year and is increasing. It is estimated to account for around 8% of global CO2 emissions.
Research and development is focusing on both CO2 reduction and CO2 sequestration, areas characterised by a range of new technologies. Key focus areas of innovation are alternative raw materials and fuels, and optimisation of cement production. Low-carbon binders could partially replace cement itself. Meanwhile, carbonatable binders can be used as a supplement that absorbs some of the CO2 otherwise released during setting.
CO2 reduction
Alternative raw materials
The search for alternative raw materials is broad, and should include consideration of both CO2 footprint and binding capabilities. One example of an alternative raw material is sewage sludge, which can also serve as a secondary fuel. Others include recycled construction materials and demolition waste, and industrial by-products such as fly ash and blast furnace slag.
Alternative raw materials - full result set
Alternative fuels
Alternative (secondary) fuels include waste material with sufficient calorimetric value which, upon sintering at high temperatures, may be fully incorporated into the cement itself. Suitable waste materials for this purpose include tyres, sewage sludge and sawdust.
Alternative fuels - full result set
Fuel reduction
There are various ways of reducing the amount of fuel used in cement production, including more efficient furnaces, heat loss reduction measures, better heat and energy recycling, and the use of by-products as components in cement production.
Fuel reduction - full result set
Low-carbon binders
Geopolymer binders can be used to replace Portland cement, and composite blends in particular are of increasing interest. These may have additional beneficial binding properties and are being continuously optimised. Alternatively, alkali-activated cements based on ground granulated blast furnace slag or fly ash may be used to produce binders with very low or zero CO2 emissions.
Low-carbon binders - full result set
Carbonatable binders
Partially replacing conventional cement with carbonatable binders reduces the CO2 emissions from limestone and also saves raw materials.
Carbonatable binders - full result set
CO2 sequestration
Supplementary cementitious materials (SCM)
Possible SCMs that can be used to help reduce emissions include suitably treated materials such as soil, waste materials, other kinds of silica and alumina-containing materials.
Supplementary cementitious materials (SCM) - full result set
Carbonated fillers or aggregates
The re-use of old cement, if suitably ground and treated, can partially replace gravel while absorbing some of the CO2 produced by concrete and may even enable a lower proportion of cement to be used.
Carbonated fillers or aggregates - full result set
Carbon dioxide hardening
CO2 itself can be introduced into mortar or concrete, for example by injection as a gas during mixing and curing. As well as using and fixing surplus CO2, this may improve the strength of concrete and therefore reduce the amount of cement required.